1.1: Our Place in Space
- Page ID
- 5647
\( \newcommand{\vecs}[1]{\overset { \scriptstyle \rightharpoonup} {\mathbf{#1}} } \)
\( \newcommand{\vecd}[1]{\overset{-\!-\!\rightharpoonup}{\vphantom{a}\smash {#1}}} \)
\( \newcommand{\id}{\mathrm{id}}\) \( \newcommand{\Span}{\mathrm{span}}\)
( \newcommand{\kernel}{\mathrm{null}\,}\) \( \newcommand{\range}{\mathrm{range}\,}\)
\( \newcommand{\RealPart}{\mathrm{Re}}\) \( \newcommand{\ImaginaryPart}{\mathrm{Im}}\)
\( \newcommand{\Argument}{\mathrm{Arg}}\) \( \newcommand{\norm}[1]{\| #1 \|}\)
\( \newcommand{\inner}[2]{\langle #1, #2 \rangle}\)
\( \newcommand{\Span}{\mathrm{span}}\)
\( \newcommand{\id}{\mathrm{id}}\)
\( \newcommand{\Span}{\mathrm{span}}\)
\( \newcommand{\kernel}{\mathrm{null}\,}\)
\( \newcommand{\range}{\mathrm{range}\,}\)
\( \newcommand{\RealPart}{\mathrm{Re}}\)
\( \newcommand{\ImaginaryPart}{\mathrm{Im}}\)
\( \newcommand{\Argument}{\mathrm{Arg}}\)
\( \newcommand{\norm}[1]{\| #1 \|}\)
\( \newcommand{\inner}[2]{\langle #1, #2 \rangle}\)
\( \newcommand{\Span}{\mathrm{span}}\) \( \newcommand{\AA}{\unicode[.8,0]{x212B}}\)
\( \newcommand{\vectorA}[1]{\vec{#1}} % arrow\)
\( \newcommand{\vectorAt}[1]{\vec{\text{#1}}} % arrow\)
\( \newcommand{\vectorB}[1]{\overset { \scriptstyle \rightharpoonup} {\mathbf{#1}} } \)
\( \newcommand{\vectorC}[1]{\textbf{#1}} \)
\( \newcommand{\vectorD}[1]{\overrightarrow{#1}} \)
\( \newcommand{\vectorDt}[1]{\overrightarrow{\text{#1}}} \)
\( \newcommand{\vectE}[1]{\overset{-\!-\!\rightharpoonup}{\vphantom{a}\smash{\mathbf {#1}}}} \)
\( \newcommand{\vecs}[1]{\overset { \scriptstyle \rightharpoonup} {\mathbf{#1}} } \)
\( \newcommand{\vecd}[1]{\overset{-\!-\!\rightharpoonup}{\vphantom{a}\smash {#1}}} \)
\(\newcommand{\avec}{\mathbf a}\) \(\newcommand{\bvec}{\mathbf b}\) \(\newcommand{\cvec}{\mathbf c}\) \(\newcommand{\dvec}{\mathbf d}\) \(\newcommand{\dtil}{\widetilde{\mathbf d}}\) \(\newcommand{\evec}{\mathbf e}\) \(\newcommand{\fvec}{\mathbf f}\) \(\newcommand{\nvec}{\mathbf n}\) \(\newcommand{\pvec}{\mathbf p}\) \(\newcommand{\qvec}{\mathbf q}\) \(\newcommand{\svec}{\mathbf s}\) \(\newcommand{\tvec}{\mathbf t}\) \(\newcommand{\uvec}{\mathbf u}\) \(\newcommand{\vvec}{\mathbf v}\) \(\newcommand{\wvec}{\mathbf w}\) \(\newcommand{\xvec}{\mathbf x}\) \(\newcommand{\yvec}{\mathbf y}\) \(\newcommand{\zvec}{\mathbf z}\) \(\newcommand{\rvec}{\mathbf r}\) \(\newcommand{\mvec}{\mathbf m}\) \(\newcommand{\zerovec}{\mathbf 0}\) \(\newcommand{\onevec}{\mathbf 1}\) \(\newcommand{\real}{\mathbb R}\) \(\newcommand{\twovec}[2]{\left[\begin{array}{r}#1 \\ #2 \end{array}\right]}\) \(\newcommand{\ctwovec}[2]{\left[\begin{array}{c}#1 \\ #2 \end{array}\right]}\) \(\newcommand{\threevec}[3]{\left[\begin{array}{r}#1 \\ #2 \\ #3 \end{array}\right]}\) \(\newcommand{\cthreevec}[3]{\left[\begin{array}{c}#1 \\ #2 \\ #3 \end{array}\right]}\) \(\newcommand{\fourvec}[4]{\left[\begin{array}{r}#1 \\ #2 \\ #3 \\ #4 \end{array}\right]}\) \(\newcommand{\cfourvec}[4]{\left[\begin{array}{c}#1 \\ #2 \\ #3 \\ #4 \end{array}\right]}\) \(\newcommand{\fivevec}[5]{\left[\begin{array}{r}#1 \\ #2 \\ #3 \\ #4 \\ #5 \\ \end{array}\right]}\) \(\newcommand{\cfivevec}[5]{\left[\begin{array}{c}#1 \\ #2 \\ #3 \\ #4 \\ #5 \\ \end{array}\right]}\) \(\newcommand{\mattwo}[4]{\left[\begin{array}{rr}#1 \amp #2 \\ #3 \amp #4 \\ \end{array}\right]}\) \(\newcommand{\laspan}[1]{\text{Span}\{#1\}}\) \(\newcommand{\bcal}{\cal B}\) \(\newcommand{\ccal}{\cal C}\) \(\newcommand{\scal}{\cal S}\) \(\newcommand{\wcal}{\cal W}\) \(\newcommand{\ecal}{\cal E}\) \(\newcommand{\coords}[2]{\left\{#1\right\}_{#2}}\) \(\newcommand{\gray}[1]{\color{gray}{#1}}\) \(\newcommand{\lgray}[1]{\color{lightgray}{#1}}\) \(\newcommand{\rank}{\operatorname{rank}}\) \(\newcommand{\row}{\text{Row}}\) \(\newcommand{\col}{\text{Col}}\) \(\renewcommand{\row}{\text{Row}}\) \(\newcommand{\nul}{\text{Nul}}\) \(\newcommand{\var}{\text{Var}}\) \(\newcommand{\corr}{\text{corr}}\) \(\newcommand{\len}[1]{\left|#1\right|}\) \(\newcommand{\bbar}{\overline{\bvec}}\) \(\newcommand{\bhat}{\widehat{\bvec}}\) \(\newcommand{\bperp}{\bvec^\perp}\) \(\newcommand{\xhat}{\widehat{\xvec}}\) \(\newcommand{\vhat}{\widehat{\vvec}}\) \(\newcommand{\uhat}{\widehat{\uvec}}\) \(\newcommand{\what}{\widehat{\wvec}}\) \(\newcommand{\Sighat}{\widehat{\Sigma}}\) \(\newcommand{\lt}{<}\) \(\newcommand{\gt}{>}\) \(\newcommand{\amp}{&}\) \(\definecolor{fillinmathshade}{gray}{0.9}\)In 2016, Pete Kostelnick ran across the country from New York to San Francisco, a distance that is one tenth the circumference of the Earth. It took him 46 days, 6 hours and 30 minutes. From the perspective of humans, the Earth is a pretty big place. However, as planets go, the Earth is small. It is worth taking a step back and reflecting on how our home planet fits into the big picture of the solar system, the galaxy, and the universe. This perspective is valuable as we consider the possibility of life elsewhere.
The scale of the solar system
We use rulers to measure the sizes of objects on our desks and miles or kilometers to measure distances to other cities. Clearly, it would not make sense to use inches to describe the distance between New York and San Francisco. Likewise, when we think about objects like planets and stars, the units of miles or kilometers are too small. We need a different measuring stick. The ideal metric will match our intuition or experience with the physical world. Some of the common ``measuring sticks'' that astronomers use are:
- Astronomical units (AU) - the distance between the Earth and the Sun - this is handy for comparing distances to planets within our solar system. One AU is 1.49 x 108 km, or 93 million miles. It takes light about 8 minutes to travel 1 AU.
- Light years (ly) - the distance that light travels in one year (note that this is not a measure of time, it is a measure of distance). This unit is handy for describing distances to stars within our galaxy. When we see the light from a star that is 40 light years away, we are seeing light that left that star 40 years ago. One light year is 9.5×1012 km or 63,240 AU.
- Parsecs (pc) - formally, this is the distance at which the orbital radius of the Earth (1 AU) subtends one arcsecond (or 1/3600 of a degree). Using parsecs as a metric for distance greatly simplifies our calculations for distances to stars that are measure with trigonometric parallaxes. One parsec also equals 3.26 light years or 206265 AU. We use parsecs for describing distances to stars in the Milky Way galaxy, and kiloparsecs (kpc = 1000 pc) or megaparsecs (Mpc = 1 million pc) for describing distances to other galaxies.
However, astronomers have the flexibility to use any handy, scale-appropriate metric. The mass or radius of a planet can be arbitrarily described in Earth units. The mass, radius and luminosity of other stars is usually given in solar units (i.e., relative to the Sun).
Consider the scale of the solar system. How does the size of the Earth compare to the size of Jupiter? or to the size of the Sun? How many Astronomical Units (AUs) from the Sun to Jupiter? What are these distances like in terms of light travel times? How long does it take a photon to travel from the Sun to the Earth?
The YouTube video below gives a great overview of the scale of the solar system.
What are your three take-away points from this video?
Where is the halfway point (spatially) between the Sun and Neptune? How does the spacing of the inner rocky planets compare to the spacing of the outer gas giants? Why might the gas giant planets be farther away from the Sun than rocky planets like Earth?
(Film by Alex Gorosh and Wylie Overstreet.)
Pointing to the stars
Astronomers use an Earth-centric coordinate system for viewing other stars. This coordinate system was inherited from the ancient (and incorrect) belief that the Earth was motionless and that everything else - the planets, stars and the Sun - revolved around the Earth. With this perspective, the sky and stars appeared to be printed onto the celestial sphere, an imaginary bubble encircling the Earth. The precise location of objects on the celestial sphere are specified by the celestial equivalent of latitude and longitude.
Some reference points are relative to the observer. The point directly overhead for the observer. The horizon is the great circle where the sky "touches" the Earth and this will also be observer-centric. The meridian is an arc that runs between the celestial poles (the projection of the Earth's north and south poles) and the zenith. The meridian is also defined relative to the observer.
However, there are absolute positions that do not depend on the location of the observer. As shown in Figure \(\PageIndex{1}\) below, the equator of the Earth is projected into space as the "celestial" equator and a second great circle, the "ecliptic" defines the orbital plane of the Earth. Because the spin axis of the Earth is tilted with respect to the orbital plane, the celestial equator is tilted relative to the ecliptic.
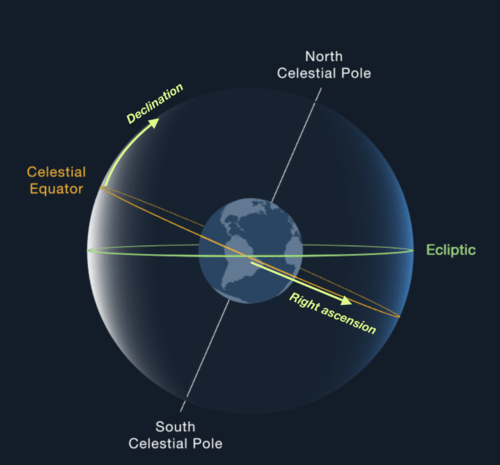
Declination is the celestial equivalent of latitude on the Earth. It ranges from 0 to 90 degrees from the celestial equator to the celestial poles. Declination is positive north of the celestial equator and negative south of the celestial equator.
Right ascension is the celestial equivalent of longitude. The zero point for right ascension is the at the intersection of the ecliptic and the celestial equator that defines the vernal equinox and the beginning of spring. This is the time of the year when the Sun is directly above the equator at noon. Right ascension ranges between 0 and 360 degrees around the celestial pole. Since the Earth rotates once every 24 hours, the sky appears to turn by 15 degrees every hour and the part of the sky that is visible is constantly changing.
Memorizing numbers?
There is no need to memorize charts of numbers, but it is worth remembering the "round number" distances to a few planets in our solar system in terms of Astronomical Units.
- Mercury is 0.4 AU from the Sun (you will learn that many of the exoplanets that have been discovered orbit much closer than 0.4 AU to their host stars).
- Jupiter is another solar system landmark at 5 AU.
- The light travel time from the Sun to the Earth is 8 minutes and the light travel time to other planets just scales with their distances. So, it takes about 40 minutes for a photon of light to travel from the Sun to Jupiter.
- The edge of our solar system? It's about 120 AU from the Sun (about 3 times farther than the distance to Pluto). Amazingly, the Voyager 1 and 2 space probes, which were launched in 1977, have left the boundary of the solar system.
Other handy numbers for the solar system:
- Jupiter is about 300 times the mass of the Earth
- The Sun is 1000 times the mass of Jupiter
- The radius of Jupiter is about 11 times the radius of Earth, but 1/10th the radius of the Sun.
NASA provides a handy fact sheet listing information about solar system planets in metric or Earth ratio units.
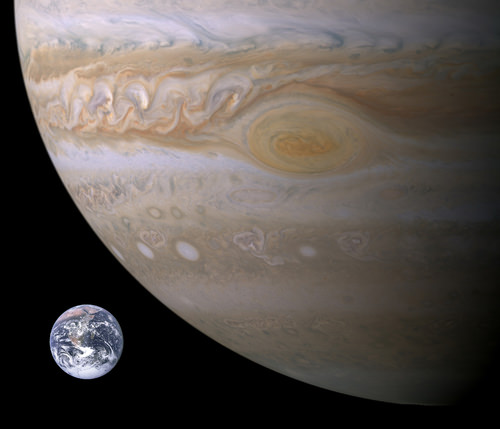
The Voyager Mission
In 1965, calculations showed that a "once in 175 year" event was about to occur: Jupiter, Saturn, Uranus and Neptune would would line up in such a way that a spacecraft could use the gravitational energy of each planet in turn to swing on to the next planet. In 1972, the Voyager mission was approved to travel to the outer solar system. On August 20, 1977, the slower Voyager II mission was launched before the faster Voyager I mission, which was launched on September 5, 1977. The Voyagers were powered by Plutonium-238 (radioactive half life of 88 years), which produced 470 W to run all of the instruments.
Voyager I flew by Jupiter, then continued on to Saturn before taking a trajectory above the ecliptic plane of the solar system planets. Voyager II used gravitational slingshots to travel from Jupiter to Saturn to Uranus and to Neptune before turning below the ecliptic plane.
The scientific discoveries from the Voyager spacecraft were stunning. Voyager I took the first image of the Earth and the moon in a single frame. Thousands of images of Jupiter were spliced together to make a movie showing the movement of storms in the atmospheres of Jupiter and Neptune. Shortly before Voyager 1 returned images of Jupiter's closest moon, Io, physicist Stanton Peale (University of California, Santa Barbara) and colleagues had published a theoretical paper, predicting that tidal energy between Jupiter and Io would cause the surface of Io to be volcanically active. Peale and his colleagues were rewarded in just a few days with the first close-up images of Io, confirming their prediction. The Voyager mission returned our first detailed images of the rings of Saturn, showing that they were comprised of icy material that is shepherded by many small Saturnian moons. Images from Voyager II showed that Jupiter, Uranus and Neptune have tenuous rings and many additional moons were discovered around these giant planets.
In addition to the rich scientific discoveries, the Voyager spacecraft also have important lessons for humanity. At a distance of 4 billion miles from the Earth, just before Voyager 1 left the solar system, Carl Sagan convinced the engineers at NASA to turn the space craft around and take a picture of the home planet. Earth appears as a pale blue dot. Upon seeing this image, Sagan wrote: "Look again at that dot. That's here. That's us. On it, everyone you love, everyone you know, everyone you ever heard of, every human being who ever was lived out their lives on this mote of dust, suspended in a sunbeam."
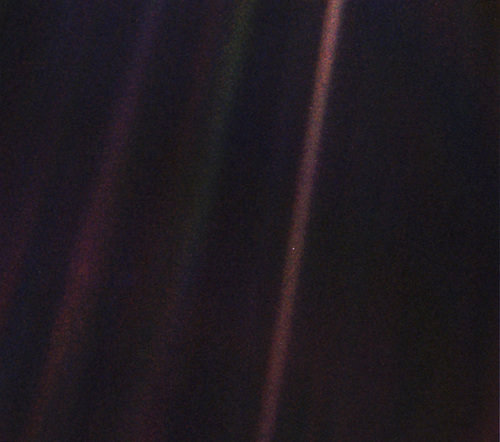
The Voyager spacecraft have both left the solar system and are now traveling in the space between the stars, the "interstellar medium." In 2012, Voyager 1 passed through the heliopause - a bubble around the Sun and planets dominated by the solar wind and Voyager 2 began passing through this boundary in 2018. The spacecraft are traveling with a speed of about 35,000 miles per hour and as of 2019, they are still beaming a weak signal back to the Earth. Because of the enormous distance, the received power of their electric chirp is about 2 Watts.

The most unique component on the Voyager probes is a time capsule of information inscribed into a gold record. A stylus for playing the record and the turning frequency are provided. Information on the record gives details about our location. The recorded information describes our biochemical make-up, including and the nucleotide bases in our DNA, and provides iconic cultural images, circa 1977. In addition to greetings in more than 50 languages, the Voyagers are carrying 90 minutes of music, including the haunting "Dark was the Night, Cold was the Ground" by Blind Willie Johnson and Chuck Berry's "Johnny B. Goode." A 1977 Saturday Night Live skit portrays extraterrestrials finding the recording and sending back the message: "Send more Chuck Berry."
The Voyager spacecraft are now destined to orbit the center of the galaxy, like all of the stars in the Milky Way. They may well live outlive our Sun and our solar system. Perhaps in the very distant future, another civilization will intercept these spacecraft. If they decode our message, they will know that once upon a time in the galaxy, there was another civilization with the generosity to send a message: "You are not alone. Here is the code for carbon-based life that lived on our planet. Even if our species is never able to learn if we are alone in the vast universe, we hope that this message will give you some answers."