12.1: Threats to Life
- Page ID
- 5644
\( \newcommand{\vecs}[1]{\overset { \scriptstyle \rightharpoonup} {\mathbf{#1}} } \)
\( \newcommand{\vecd}[1]{\overset{-\!-\!\rightharpoonup}{\vphantom{a}\smash {#1}}} \)
\( \newcommand{\id}{\mathrm{id}}\) \( \newcommand{\Span}{\mathrm{span}}\)
( \newcommand{\kernel}{\mathrm{null}\,}\) \( \newcommand{\range}{\mathrm{range}\,}\)
\( \newcommand{\RealPart}{\mathrm{Re}}\) \( \newcommand{\ImaginaryPart}{\mathrm{Im}}\)
\( \newcommand{\Argument}{\mathrm{Arg}}\) \( \newcommand{\norm}[1]{\| #1 \|}\)
\( \newcommand{\inner}[2]{\langle #1, #2 \rangle}\)
\( \newcommand{\Span}{\mathrm{span}}\)
\( \newcommand{\id}{\mathrm{id}}\)
\( \newcommand{\Span}{\mathrm{span}}\)
\( \newcommand{\kernel}{\mathrm{null}\,}\)
\( \newcommand{\range}{\mathrm{range}\,}\)
\( \newcommand{\RealPart}{\mathrm{Re}}\)
\( \newcommand{\ImaginaryPart}{\mathrm{Im}}\)
\( \newcommand{\Argument}{\mathrm{Arg}}\)
\( \newcommand{\norm}[1]{\| #1 \|}\)
\( \newcommand{\inner}[2]{\langle #1, #2 \rangle}\)
\( \newcommand{\Span}{\mathrm{span}}\) \( \newcommand{\AA}{\unicode[.8,0]{x212B}}\)
\( \newcommand{\vectorA}[1]{\vec{#1}} % arrow\)
\( \newcommand{\vectorAt}[1]{\vec{\text{#1}}} % arrow\)
\( \newcommand{\vectorB}[1]{\overset { \scriptstyle \rightharpoonup} {\mathbf{#1}} } \)
\( \newcommand{\vectorC}[1]{\textbf{#1}} \)
\( \newcommand{\vectorD}[1]{\overrightarrow{#1}} \)
\( \newcommand{\vectorDt}[1]{\overrightarrow{\text{#1}}} \)
\( \newcommand{\vectE}[1]{\overset{-\!-\!\rightharpoonup}{\vphantom{a}\smash{\mathbf {#1}}}} \)
\( \newcommand{\vecs}[1]{\overset { \scriptstyle \rightharpoonup} {\mathbf{#1}} } \)
\( \newcommand{\vecd}[1]{\overset{-\!-\!\rightharpoonup}{\vphantom{a}\smash {#1}}} \)
\(\newcommand{\avec}{\mathbf a}\) \(\newcommand{\bvec}{\mathbf b}\) \(\newcommand{\cvec}{\mathbf c}\) \(\newcommand{\dvec}{\mathbf d}\) \(\newcommand{\dtil}{\widetilde{\mathbf d}}\) \(\newcommand{\evec}{\mathbf e}\) \(\newcommand{\fvec}{\mathbf f}\) \(\newcommand{\nvec}{\mathbf n}\) \(\newcommand{\pvec}{\mathbf p}\) \(\newcommand{\qvec}{\mathbf q}\) \(\newcommand{\svec}{\mathbf s}\) \(\newcommand{\tvec}{\mathbf t}\) \(\newcommand{\uvec}{\mathbf u}\) \(\newcommand{\vvec}{\mathbf v}\) \(\newcommand{\wvec}{\mathbf w}\) \(\newcommand{\xvec}{\mathbf x}\) \(\newcommand{\yvec}{\mathbf y}\) \(\newcommand{\zvec}{\mathbf z}\) \(\newcommand{\rvec}{\mathbf r}\) \(\newcommand{\mvec}{\mathbf m}\) \(\newcommand{\zerovec}{\mathbf 0}\) \(\newcommand{\onevec}{\mathbf 1}\) \(\newcommand{\real}{\mathbb R}\) \(\newcommand{\twovec}[2]{\left[\begin{array}{r}#1 \\ #2 \end{array}\right]}\) \(\newcommand{\ctwovec}[2]{\left[\begin{array}{c}#1 \\ #2 \end{array}\right]}\) \(\newcommand{\threevec}[3]{\left[\begin{array}{r}#1 \\ #2 \\ #3 \end{array}\right]}\) \(\newcommand{\cthreevec}[3]{\left[\begin{array}{c}#1 \\ #2 \\ #3 \end{array}\right]}\) \(\newcommand{\fourvec}[4]{\left[\begin{array}{r}#1 \\ #2 \\ #3 \\ #4 \end{array}\right]}\) \(\newcommand{\cfourvec}[4]{\left[\begin{array}{c}#1 \\ #2 \\ #3 \\ #4 \end{array}\right]}\) \(\newcommand{\fivevec}[5]{\left[\begin{array}{r}#1 \\ #2 \\ #3 \\ #4 \\ #5 \\ \end{array}\right]}\) \(\newcommand{\cfivevec}[5]{\left[\begin{array}{c}#1 \\ #2 \\ #3 \\ #4 \\ #5 \\ \end{array}\right]}\) \(\newcommand{\mattwo}[4]{\left[\begin{array}{rr}#1 \amp #2 \\ #3 \amp #4 \\ \end{array}\right]}\) \(\newcommand{\laspan}[1]{\text{Span}\{#1\}}\) \(\newcommand{\bcal}{\cal B}\) \(\newcommand{\ccal}{\cal C}\) \(\newcommand{\scal}{\cal S}\) \(\newcommand{\wcal}{\cal W}\) \(\newcommand{\ecal}{\cal E}\) \(\newcommand{\coords}[2]{\left\{#1\right\}_{#2}}\) \(\newcommand{\gray}[1]{\color{gray}{#1}}\) \(\newcommand{\lgray}[1]{\color{lightgray}{#1}}\) \(\newcommand{\rank}{\operatorname{rank}}\) \(\newcommand{\row}{\text{Row}}\) \(\newcommand{\col}{\text{Col}}\) \(\renewcommand{\row}{\text{Row}}\) \(\newcommand{\nul}{\text{Nul}}\) \(\newcommand{\var}{\text{Var}}\) \(\newcommand{\corr}{\text{corr}}\) \(\newcommand{\len}[1]{\left|#1\right|}\) \(\newcommand{\bbar}{\overline{\bvec}}\) \(\newcommand{\bhat}{\widehat{\bvec}}\) \(\newcommand{\bperp}{\bvec^\perp}\) \(\newcommand{\xhat}{\widehat{\xvec}}\) \(\newcommand{\vhat}{\widehat{\vvec}}\) \(\newcommand{\uhat}{\widehat{\uvec}}\) \(\newcommand{\what}{\widehat{\wvec}}\) \(\newcommand{\Sighat}{\widehat{\Sigma}}\) \(\newcommand{\lt}{<}\) \(\newcommand{\gt}{>}\) \(\newcommand{\amp}{&}\) \(\definecolor{fillinmathshade}{gray}{0.9}\)For all of us, even the Earth, and even the Sun, the end is coming. Here we describe what may be in the future for our Solar System and our Sun and what it will mean for life on Earth.
Near-Earth Asteroids
Yes, there's an app for that! There is even a twitter that tracks and monitors the location and trajectories of near-Earth asteroids (NEAs), asteroids that cross or closely approach the Earth's orbit. Asteroids are rocky debris left over from the formation of the Solar System and are likely to be a component of extrasolar systems as well. Most orbit in a "belt" located between Mars and Jupiter where there are millions of asteroids varying in sizes from 1 km to hundreds of kilometers.
Potentially hazardous asteroids (PHA) have been with us for millions of years, weaving in and out of Earth's orbital path. Classification as a PHA depends on the three things: 1) mass, 2) material strength, and 3) location. The mass determines the amount of force the asteroid will impart if it collides with the Earth. The material strength determines how well the asteroid will survive through the Earth's protective atmosphere, in which many meteoroids burn up. Location determines if the asteroid will collide with the Earth at all. These three things together determine how likely it is for an asteroid to survive to hit the Earth and cause great damage.
Detection campaigns work to spot comets or PHAs against background stars. Further away stars provide an inert frame of reference, allowing closer, moving objects to be distinguished. As of October 2016, approximately 15,000 near-Earth asteroids have been found, with an average of 30 new asteroids discovered each week. No, no, do not panic yet. Only a few are hazardous for Earth and they are all being kept close track of.
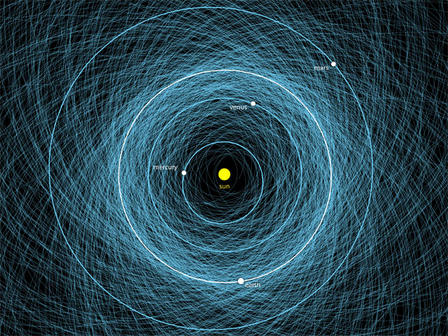
There are three classes of NEAs. Apollo asteroids have semi-major axes larger than 1 AU, the semi-major axis of the Earth. These make up about 62% of all NEAs, including the asteroids that made Earth-crossing orbits in December 2019. Aten asteroids, making up just 6% of NEAs, have a semi-major axes of smaller than 1 AU. Amor asteroids (32%) merely approach Earth's orbit, but crosses orbits with asteroids that orbit between the Earth and Mars.
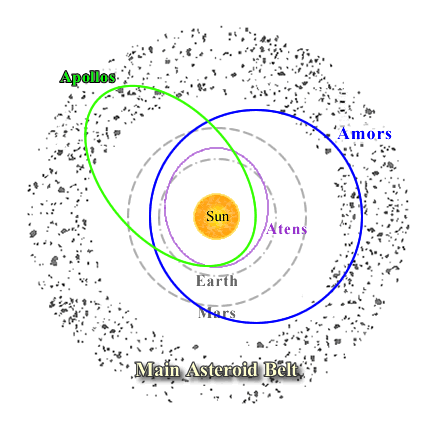
Impact probes are used to better understand the strength and composition of asteroids. These probes are sent to crash into asteroids, causing material from the asteroid to vaporize around the probe, which can then be analyzed. Probes can also measure the water content of asteroids, which gives a clue to the water abundance in the Solar System. It is theorized that much of the water on Earth was once delivered to Earth through asteroids.
In 1908, the Tunguska asteroid exploded over Siberia with a force comparable to 10-15 megatons of TNT. Thought to measure 120 m across, the explosion fortunately occurred over a largely uninhabited area. Some settlers reported seeing a flash and a large explosion as the asteroid burst in the air about the ground. Shock waves knocked down millions of trees in a 800 mile radius and registered a 5.0 on the Richter scale. Now, 100 years later, the trees are only just beginning to recover from the explosion.
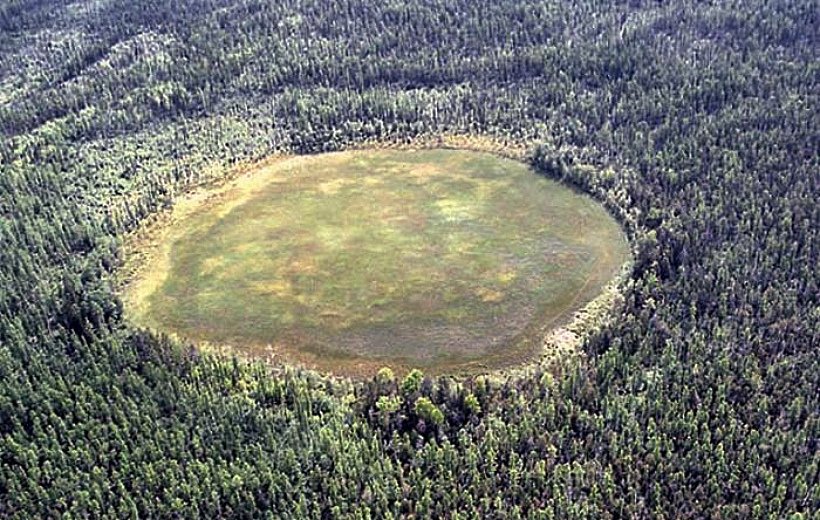
Russia was hit again in February 2013 when a 20 m wide meteor crashed down with the force of 500 kilotons of TNT. Shock waves from the explosion shattered windows in hundreds of buildings. The explosion itself was hot and bright enough to cause skin and retinal burns.
Asteroid impacts release substantial amounts of collision energy and can cause the type of devastation witness by all the now super-dead dinosaurs of the Cretaceous period. What then, are the real chances that the Earth, or any other habitable planet, is hit by an asteroid capable of that type of global destruction?
Every day, earth is bombarded by more than 100 tons of dust and sand-sized particles from space that burn up in the atmosphere. Roughly once per year, an automobile-sized asteroid will hit the Earth's atmosphere. It will create a glowing fireball in the atmosphere, but at this size, the asteroid will still burn up before reaching Earth's surface. From calculations, we know that roughly every 2,000 years, an asteroid larger than the size of a football filed could hit the Earth and cause significant local damage. Only every million years or so will there be an object with large enough mass to threaten the Earth's entire civilization.
Is there no hope? No, yes there is hope. There are a number of proposals on how to deal with approaching asteroids. We could always blow up the asteroid, but it will be hard to mitigate the debris from such an attack, and we currently only have the resources to dispel an asteroid of about 1 km in diameter. The Treaty on Principles Governing the Activities of States in the Exploration and Other Uses of Outer Space disallows the use of nuclear weapons in space.
Other suggestions propose changing the orbit of approaching asteroids just enough so that they are no longer on a collision course with the Earth. This could be done using a gravity tractor, solar sails, or by attaching a ballast to the asteroid that will change its center of mass just enough to change its trajectory. It may also be possible to use concentrated lasers to vaporize part of an incoming asteroid. The vaporized areas of the rock will let off plumes of super-heated gases and debris that will have the same effect of a rocket and propel the asteroid in a different direction. While certainly threatening, asteroids pose only an occasional threat to life and perhaps not a threat at all to advanced enough civilizations.
Domesticated Asteroids
While it seems most people would agree that the only good asteroid is a far away asteroid, others have proposed sending out a probe to capture an asteroid and bring it back. It could be parked near the moon and enable astronauts to regularly go and examine what is usually so inaccessible. In July 2014, NASA sent out a call of proposals for ideas on how to accomplish such a mission and received over 400 proposals from private companies to international organizations!
Solar System Stability
Planets are kept in their orbits primarily through gravitational interactions between the planet and their host star. However, planets also feel a much smaller but non-negligible gravitational pull from the other planets in the system. Though the planets in our Solar System are relatively well separated, this is not always the case in other Solar Systems, and may not even always be the case for our own system. The closer two bodies are too each other, the stronger their gravitational pull will be on each other.
Close encounters between planets can affect their orbits' eccentricities, semi-major axes, and the relative inclination. These effects are accentuated in the case of resonant systems. Resonance describes the situation in which two planets have periods that form an integer ratio. For example, if one planet has a period of two years and a second planet has a period of four years, these two planets are said to be in resonance. Planets in resonance will always approach each other at the same point in their orbit. This means their strongest gravitational interactions will always occur at the same point and become additive. Today, we can use computer simulations to determine what will happen to different systems of planets as they evolve through time that will expose these types of interactions.
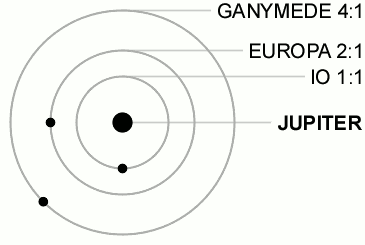
Planets may also move around during formation. This may push smaller, more-likely rocky planets into the host star, or send debris like asteroids and comets into the path of other planets. Such motion in our Solar System is thought to have caused the period of late heavy bombardment, which left its mark on our Moon. This type of mechanism may have caused the strange position of hot Jupiters, which are commonly found and much closer in than where they could have formed.
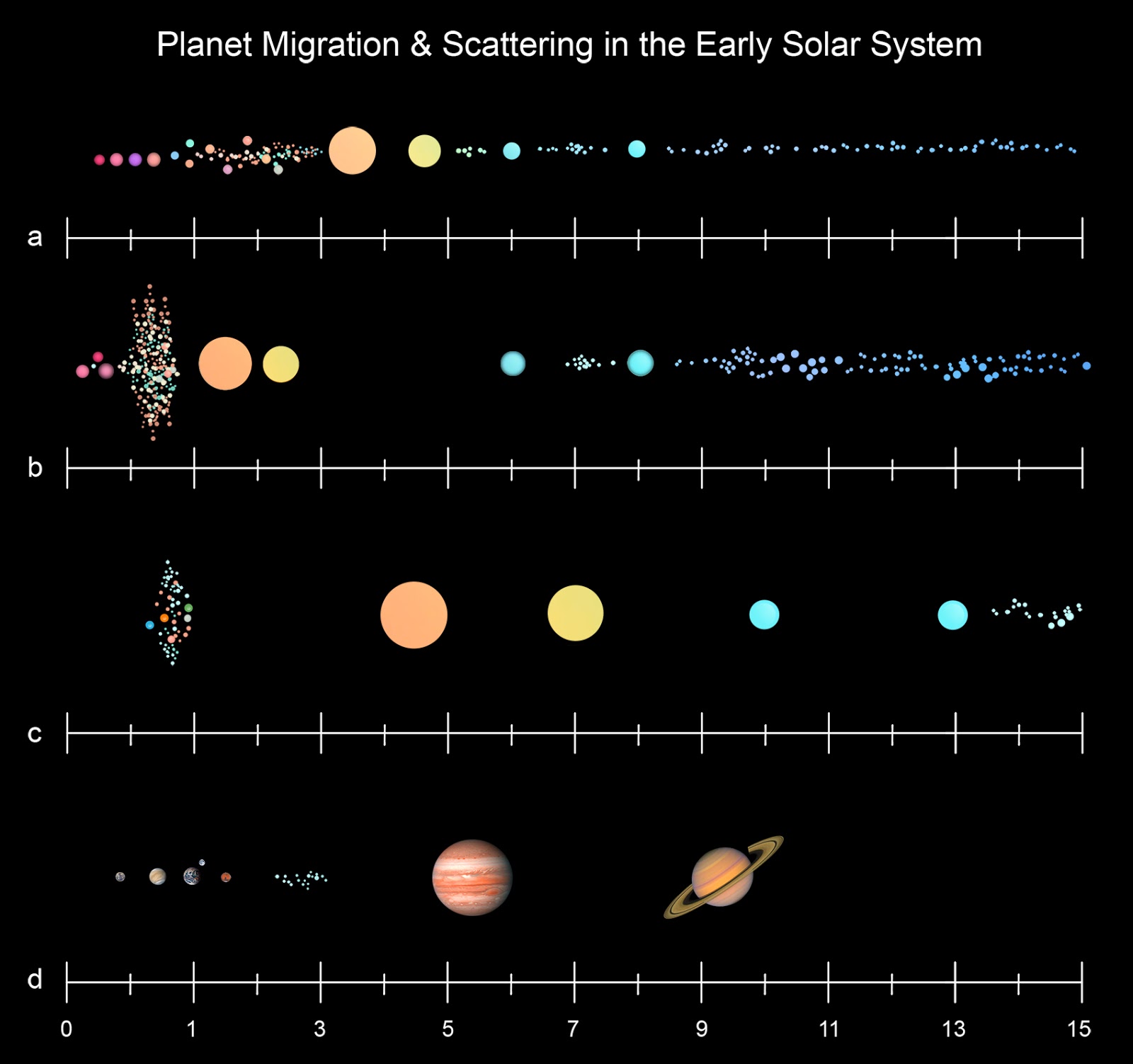
In 2008, a paper by Batygin and Laughlin showed that on million year time scales, planetary orbits may evolve in random, but ultimately stable ways. However, on longer timescales, orbits can rapidly and spontaneously evolve into chaotic states hard for even the best computer simulations to predict. Subtle interactions between the different planets and objects with mass in the solar system are capable of enacting significant change over the dynamical state of a system of planets. Close encounters will amplify these changes, rearrange orbits, and eject small bodies out of the Solar System. Resonant affects could even depopulate entire areas in a planetary system. The architecture and stability of a planetary system are important contributors to a planet's potential habitability.
Solar Evolution
The Sun, the light of our lives, formed 4.6 billion years ago and will last in its current state for about 5 billion more years. Recall that the Sun is fueled by nuclear reactions in its core that fuse hydrogen atoms to form helium and energy. This makes it a main-sequence star. These reaction serve to generate the outward pressure that balances with the Sun's gravity which pulls inwards.
In 5 billion years, the sun will run out of the hydrogen it needs to fuel this outwards push. It will instead begin to combine helium atoms to make carbon atoms, which will release far more energy. The Sun will begin to expand and blow up into a red giant, whose radius will be approximately the orbit of the Earth.
Ultimately, the Sun will also run out of helium to burn. It will eject its outer envelope of hot, charged plasma, which is misleadingly named a planetary nebula. What remains is a white dwarf, which still has the approximate mass of the once great Sun, but is about the size of Earth. The incredibly dense white dwarf is only held up by electron pressure, generated from the inability of electrons to occupy too close a space with one another.

Distant planets may continue to orbit the dead stars since the mass of the star remains nearly constant, but planets within the orbit of Earth are not similarly saved. As a red giant, the Sun will expand nearly to the Earths orbit. Close-in planets, such as Mercury and Venus, will become engulfed within the Sun itself. If the Sun does not engulf the Earth, it will certainly irradiate the Earth with an excessive amount of high-energy radiation that no amount of sunscreen can withstand. The different mass distribution of the Sun throughout its different evolutionary stages may also play significant havoc on close-in orbits. The habitability of close-in planets is strongly upper limited by the time its host star remains on the main sequence, which is determined by the mass of the star.
Planet Eaters
White dwarfs no longer have nuclear fusion providing an outward force. This causes heavier elements to settle down to the core of a white dwarf due to the effects of gravity (like how sediment will settle in a glass if it is kept still). Therefore, white dwarfs should have only the lightest element, hydrogen, on their surfaces.
In 1917, astronomer vanMaanen found a white dwarf that showed traces of oxygen, magnesium, silicon, and iron on its surface, elements that commonly indicate the presence of rocks. This may well have been the first discovered exoplanet - or rather, the remains of an exoplanet plastered onto the surface of its once hospitable host star.