8.2: Early Evolution of Microbial Life
- Page ID
- 5665
\( \newcommand{\vecs}[1]{\overset { \scriptstyle \rightharpoonup} {\mathbf{#1}} } \)
\( \newcommand{\vecd}[1]{\overset{-\!-\!\rightharpoonup}{\vphantom{a}\smash {#1}}} \)
\( \newcommand{\id}{\mathrm{id}}\) \( \newcommand{\Span}{\mathrm{span}}\)
( \newcommand{\kernel}{\mathrm{null}\,}\) \( \newcommand{\range}{\mathrm{range}\,}\)
\( \newcommand{\RealPart}{\mathrm{Re}}\) \( \newcommand{\ImaginaryPart}{\mathrm{Im}}\)
\( \newcommand{\Argument}{\mathrm{Arg}}\) \( \newcommand{\norm}[1]{\| #1 \|}\)
\( \newcommand{\inner}[2]{\langle #1, #2 \rangle}\)
\( \newcommand{\Span}{\mathrm{span}}\)
\( \newcommand{\id}{\mathrm{id}}\)
\( \newcommand{\Span}{\mathrm{span}}\)
\( \newcommand{\kernel}{\mathrm{null}\,}\)
\( \newcommand{\range}{\mathrm{range}\,}\)
\( \newcommand{\RealPart}{\mathrm{Re}}\)
\( \newcommand{\ImaginaryPart}{\mathrm{Im}}\)
\( \newcommand{\Argument}{\mathrm{Arg}}\)
\( \newcommand{\norm}[1]{\| #1 \|}\)
\( \newcommand{\inner}[2]{\langle #1, #2 \rangle}\)
\( \newcommand{\Span}{\mathrm{span}}\) \( \newcommand{\AA}{\unicode[.8,0]{x212B}}\)
\( \newcommand{\vectorA}[1]{\vec{#1}} % arrow\)
\( \newcommand{\vectorAt}[1]{\vec{\text{#1}}} % arrow\)
\( \newcommand{\vectorB}[1]{\overset { \scriptstyle \rightharpoonup} {\mathbf{#1}} } \)
\( \newcommand{\vectorC}[1]{\textbf{#1}} \)
\( \newcommand{\vectorD}[1]{\overrightarrow{#1}} \)
\( \newcommand{\vectorDt}[1]{\overrightarrow{\text{#1}}} \)
\( \newcommand{\vectE}[1]{\overset{-\!-\!\rightharpoonup}{\vphantom{a}\smash{\mathbf {#1}}}} \)
\( \newcommand{\vecs}[1]{\overset { \scriptstyle \rightharpoonup} {\mathbf{#1}} } \)
\( \newcommand{\vecd}[1]{\overset{-\!-\!\rightharpoonup}{\vphantom{a}\smash {#1}}} \)
\(\newcommand{\avec}{\mathbf a}\) \(\newcommand{\bvec}{\mathbf b}\) \(\newcommand{\cvec}{\mathbf c}\) \(\newcommand{\dvec}{\mathbf d}\) \(\newcommand{\dtil}{\widetilde{\mathbf d}}\) \(\newcommand{\evec}{\mathbf e}\) \(\newcommand{\fvec}{\mathbf f}\) \(\newcommand{\nvec}{\mathbf n}\) \(\newcommand{\pvec}{\mathbf p}\) \(\newcommand{\qvec}{\mathbf q}\) \(\newcommand{\svec}{\mathbf s}\) \(\newcommand{\tvec}{\mathbf t}\) \(\newcommand{\uvec}{\mathbf u}\) \(\newcommand{\vvec}{\mathbf v}\) \(\newcommand{\wvec}{\mathbf w}\) \(\newcommand{\xvec}{\mathbf x}\) \(\newcommand{\yvec}{\mathbf y}\) \(\newcommand{\zvec}{\mathbf z}\) \(\newcommand{\rvec}{\mathbf r}\) \(\newcommand{\mvec}{\mathbf m}\) \(\newcommand{\zerovec}{\mathbf 0}\) \(\newcommand{\onevec}{\mathbf 1}\) \(\newcommand{\real}{\mathbb R}\) \(\newcommand{\twovec}[2]{\left[\begin{array}{r}#1 \\ #2 \end{array}\right]}\) \(\newcommand{\ctwovec}[2]{\left[\begin{array}{c}#1 \\ #2 \end{array}\right]}\) \(\newcommand{\threevec}[3]{\left[\begin{array}{r}#1 \\ #2 \\ #3 \end{array}\right]}\) \(\newcommand{\cthreevec}[3]{\left[\begin{array}{c}#1 \\ #2 \\ #3 \end{array}\right]}\) \(\newcommand{\fourvec}[4]{\left[\begin{array}{r}#1 \\ #2 \\ #3 \\ #4 \end{array}\right]}\) \(\newcommand{\cfourvec}[4]{\left[\begin{array}{c}#1 \\ #2 \\ #3 \\ #4 \end{array}\right]}\) \(\newcommand{\fivevec}[5]{\left[\begin{array}{r}#1 \\ #2 \\ #3 \\ #4 \\ #5 \\ \end{array}\right]}\) \(\newcommand{\cfivevec}[5]{\left[\begin{array}{c}#1 \\ #2 \\ #3 \\ #4 \\ #5 \\ \end{array}\right]}\) \(\newcommand{\mattwo}[4]{\left[\begin{array}{rr}#1 \amp #2 \\ #3 \amp #4 \\ \end{array}\right]}\) \(\newcommand{\laspan}[1]{\text{Span}\{#1\}}\) \(\newcommand{\bcal}{\cal B}\) \(\newcommand{\ccal}{\cal C}\) \(\newcommand{\scal}{\cal S}\) \(\newcommand{\wcal}{\cal W}\) \(\newcommand{\ecal}{\cal E}\) \(\newcommand{\coords}[2]{\left\{#1\right\}_{#2}}\) \(\newcommand{\gray}[1]{\color{gray}{#1}}\) \(\newcommand{\lgray}[1]{\color{lightgray}{#1}}\) \(\newcommand{\rank}{\operatorname{rank}}\) \(\newcommand{\row}{\text{Row}}\) \(\newcommand{\col}{\text{Col}}\) \(\renewcommand{\row}{\text{Row}}\) \(\newcommand{\nul}{\text{Nul}}\) \(\newcommand{\var}{\text{Var}}\) \(\newcommand{\corr}{\text{corr}}\) \(\newcommand{\len}[1]{\left|#1\right|}\) \(\newcommand{\bbar}{\overline{\bvec}}\) \(\newcommand{\bhat}{\widehat{\bvec}}\) \(\newcommand{\bperp}{\bvec^\perp}\) \(\newcommand{\xhat}{\widehat{\xvec}}\) \(\newcommand{\vhat}{\widehat{\vvec}}\) \(\newcommand{\uhat}{\widehat{\uvec}}\) \(\newcommand{\what}{\widehat{\wvec}}\) \(\newcommand{\Sighat}{\widehat{\Sigma}}\) \(\newcommand{\lt}{<}\) \(\newcommand{\gt}{>}\) \(\newcommand{\amp}{&}\) \(\definecolor{fillinmathshade}{gray}{0.9}\)Types of Life
The first instance of life was likely very simple and single-celled. Today, life is fantastically varied and complex. We separate the life we see today into three domains based on shared cellular structure and genetic material. The three domains of life, archaea, bacteria, and eukaryotes, are a diversification of the Last Universal Common Ancestor.

- Bacteria make up the largest domain with both the greatest number of individual species, and a biomass that exceeds the combined biomass of all plants and animals. Bacteria were one of the earliest forms of life on our planet and they are found in most habitats. Bacteria can rapidly recombine their genes with other bacteria to allow for genetic innovations, such as resistance to antibiotics.
- Archaea are genetically distinct from bacteria with their own, separate domain. Archaea are known to generate energy in a variety of ways and have been found in some of the most extreme environments on Earth.
- Eukaryotes are distinct from prokaryotes in containing a central nucleus enclosed in a membrane and also contain other membrane-bound organelles. Examples of eukaryote organelles include chloroplast, the site of photosynthesis in plants and some algae, and mitochondria, where energy is generated in a cell. Eukaryotes can be uni- or multi-cellular, allowing for larger and more complex organisms.

Both bacteria and archaea are prokaryotes: single-celled microbes that do not contain membrane-bound organelles. However, the membranes in archaea incorporate a different type of lipid than either bacteria or eukaryotes. Genetic analysis reveals that archaea are closer to eukaryotes in an evolutionary sense than they are to Bacteria. Archaea and eukaryotes use many of the same enzymes for DNA translation.
Endosymbiosis
Eukaryotic cells have gained membrane-bound organelles and increased complexity through the process of endosymbiosis. Endosymbiosis is a process whereby primitive organisms benefited by living inside other organisms. Chloroplasts and mitochondria are examples of highly complicated organelles in eukaryotes that have their own membrane. They retain the DNA, messenger RNA, transfer RNA and ribosomes of their bacterial ancestors before they were symbiotically incorporated into larger eukaryotic cells. The larger cell presumably provided protection and easy access to organic molecules while the chloroplast and mitochondria provided energy to the larger cell. This beneficial relationship led to the creation of larger, more efficient cells.
Mother's Mitochondria
With sexual reproduction, the offspring ends up with a combination of the mother and father's DNA. However, the DNA found in mitochondria is exclusively the mother's DNA. When cells are replicated, the mitochondria split themselves as needed and so maintain a self-consistent set of DNA. With mammals, the egg destroys most of the mitochondria in sperm when they merge. In addition, most of the sperm's mitochondria is positioned in the tail to provide energy and does not make it into the egg.
This preservation of maternal mitochondrial DNA is often used to trance ancestries. Because it is contained only in the mitochondria and is infrequently used, mitochondrial DNA also suffers less mutations. It is therefore also helpful in determining the ancestry of different species and how they fit onto the tree of life.
Cyanobacteria
The rise of oxygen likely began with organisms known as cyanobacteria, an early type of photosynthetic bacteria thought to be the first organism to produce oxygen as a byproduct. Photosynthesis is the process by which organisms can harness the energy of the sun to generate energy for their own use. Cyanobacteria are the only know prokaryotes to produce oxygen and this adaptation brought about the destruction of many other organisms.
Oxidation Reactions and the Free Radicals
Oxidation describes a chemical process in which a molecule, atom, or ion loses an electron. Oxygen is a particularly good oxidizing agent because its nucleus strongly attracts electrons to fill its valence shell. Oxidation often produces free radicals, which are very reactive. They can attack and break apart bonds in other molecules, inciting a chain reaction that is damaging for biochemical reactions.
Antioxidants are compounds that inhibit oxidation and thereby prevent the formation of free radicals. Plants and animals have many natural antioxidant systems in place to guard against this or use antioxidant vitamins such as vitamin A, vitamin C, and vitamin E. A certain amount of antioxidants are required in a well-balanced diet. However, clinical studies have been unable to prove benefits of artificially increasing antioxidant intake or antioxidant supplements.
The rise in atmospheric oxygen was far from smooth and steady (figure below). Cyanobacteria slowly become more abundant, but there was still a significant delay in the build up of oxygen. There are several processes that would have hindered the rise in oxygen. Oxygen would have reacted with various chemicals, mainly iron, in the oceans and these reactions would trap oxygen, prevent it from building up the atmosphere.
The amount of oxygen in the atmosphere as a function of billions of years ago (Gyr). While the start of photosynthesis corresponds to an increase an oxygen, there is a notable plateau before oxygen jumps up to current day levels.
Oxygen may also have taken up by microbes in metabolic pathways that generate energy. Organisms that used the oxygen to oxidize ammonia appear to have been plentiful at the time and could have been effective in the reduction of free-floating oxygen.
Other organisms produced methane as a byproduct that could have acted as a sink for atmospheric oxygen. However, organisms that are known to produce methane require nickel to carry out the necessary reactions, and concentrations of nickel were dropping. The decrease of nickel would mean less excreted methane, and would allow oxygen to begin accumulating.
The increase of oxygen in the atmosphere was poisonous for anaerobic organisms. Most life before the Great Oxidation Event was anaerobic, so the rise of atmospheric oxygen surely resulted in one of the most significant extinction events in Earth's history. The fossil record shows a mass extinction of anaerobic life around 2.4-1.6 billion years ago, coincident with the rise of aerobic life.
Anaerobic vs Aerobic
The rise of atmospheric oxygen coincided with the appearance of far more complicated life forms. There is every reason to believe that the rise in oxygen would have responsible since aerobic metabolisms are more efficient.
As David Bowie sang: Ch-Ch-Ch-Ch-Changes. This is a key attribute of life!
Respiration, in biological terms, describes the process by which organisms convert nutrients into usable energy by forming ATP bonds. Respiration begins with glycolysis, wherein glucose, a sugar, is broken down to form two molecules of a compound called pyruvate along with two molecules of ATP. Without oxygen, organisms have no choice but to undergo anaerobic respiration, or fermentation. Anaerobic respiration of yeast is what makes bread rise and beer bubbly. In this scenario, pyruvate is shuttled down a pathway which produces just two molecules of ATP.
Sore Winners
A byproduct of anaerobic respiration is a molecule called lactic acid. Lactic acid should be very familiar to any athlete or any student who has been very late to class and had to make a run for it. When our bodies overexert themselves, we begin to use up more oxygen than we are can take in. In order to produce the energy needed to keep running, the body switches to anaerobic respiration, which leads to a build up of lactic acid. Lactic acid can damage muscle cells and hinder recovery. The process of producing this lactate is also the cause of next-day muscle soreness.
In the presence of oxygen, aerobic respiration becomes possible. With the help of oxygen, pyruvate can be broken down and enters a more complicated pathway known as the Krebs Cycle or the Citric Acid Cycle (Note that organisms exhibit an enormous variety of metabolic pathways; the citric acid cycle merely represents one of the more common and well understood pathways). Through the Citric Acid Cycle, organisms can produce from 30 to 36 ATP from just one molecule of glucose. Though more complicated to assemble, and therefore likely taking longer to evolve, this process can be up to 18-fold more efficient than anaerobic respiration. With more energy, it is possible to carry out more biochemical processes. This would have allowed cells to become increasingly complex and trend towards the more varied, multicellular life we see today. With great metabolism comes great complexity.
Viruses?
Viruses are even more abundant than bacteria. A virus consists of three functional parts: (1) genetic material, (2) protein coat, and typically (3) an envelope of lipids outside the protein coat. For genetic material, viruses have been discovered to use both DNA and RNA. The protein coat, also known as the capsid, encases and protects this genetic material. The envelope of lipids adds an additional layer of protection.
However, the question of whether viruses can be considered life is hotly debated. Viruses have their own genetic material and are even capable of evolving through natural selection. Viruses survive and replicate by infecting a host cell. After attaching to a cell, the virus injects its DNA into the host cell. Now, the virus is capable of taking over the host cell's replication mechanisms. In doing so, the virus can now create copies of itself until it has exhausted the cell's resources. Millions of viruses can be made in this period before the cell dies and viruses escape to infect new host cells. Because viruses require the metabolism of a host cell to produce energy and reproduce, they can not be called self-sustaining. In this way, viruses fail the NASA definition of life.
Despite their differences, evidence of viruses appear wherever life does, suggesting that viruses and life evolved together. The history of viruses is traced through their DNA or RNA and has given rise to three theories on how viruses came to be.
Regressive evolution theory proposes that viruses were once components of small, parasitic cells. Similar to the idea of endosymbiosis, viruses may have begun as small structures within larger cells that over time became separated. In fact, there exists today some bacteria that, like viruses, can only reproduce in a host cell. As they evolved, these small parasitic structures regressed further from cell-like characteristics, becoming the viruses we see today. However, there is no evidence of types of cells today that could serve as an intermediary between early and present day viruses. Even the smallest cellular parasites fail to really resemble viruses at all.
Another theory is the escaped gene theory, which proposes that viruses got their start as DNA or RNA that escaped from the genome of a larger organism. Surprisingly mobile DNA has recently come to play a large role in biology. Plasmids (figure below), are circular units of DNA and separate from the genome of an organism. They are most commonly found in bacteria and have been known to move between cells. Scientists have also recently discovered transposons, or "jumping genes", which are large segments of DNA that can move around within a cell's genome. While either of these mechanisms could have provided the genetic material for viruses, it remains unclear where the complicated capsids enclosing these genes arose from.
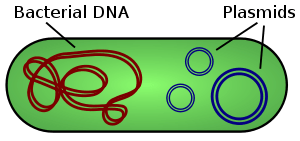
The pre-cellular origin theory proposes that viruses formed from complex proteins that spontaneously arose even before RNA. Viruses could have evolved along side the first cells or even before. However, this fails to account for the dependency viruses have on host cells. If viruses need the mechanism of a host cell to survive, how did they manage before cells even evolved?
The exact origin of viruses, much like the origin of life, remains an open question. This vein of research is being pushed forward through analysis of viral and host DNA sequences. What we can say is that genetic comparisons show that the origins of viruses may have predated life splitting into the three different domains. Perhaps the precursors to viruses evolved from the self-replicating molecules that dominated the RNA world. Similar to RNA, viruses are capable of self-assembling in host cells.
Viruses are now well enough understood to be used as tools in biomedical research. In a process called gene therapy, researchers use viruses to inserting genes into specific cells, offering possible treatments for diseases like cystic fibrosis. Some viruses will seek out and destroy cancer cells, while leaving healthy cells alone, allowing for a highly targeted and effective treatments.